General aspects of the immune response against nanocarriers
The immune response against nanocarriers occurs in a similar way to microorganisms, including viruses and bacteria. As expected, the complexity of this response is high and depends on several factors.
The immune response begins immediately after transposing physical and chemical barriers, such as:
- the skin;
- mucous membranes;
- the tear;
- gastric juice;
- secretions in general.
These barriers are a challenge for nanocarriers whose route of administration is intranasal, intrapulmonary, oral, and others. Intravenous formulations are an exception to this rule, as they directly reach the bloodstream.
Once in the body – whether in the skin, lungs, blood, or other organs and tissues – the nanocarriers are recognized by the proteins and cells of the immune system. A set of proteins, including those from the complement cascade, adhere to the surface of the nanocarriers forming a cover called the protein crown.
The complement cascade comprises approximately 50 proteins present in biological fluids or inserted into cells, whose functions are:
- mark invading microorganisms for recognition by immune cells – an activity known as opsonization;
- attracting cells from the immune system (such as granulocytes, monocytes, macrophages, dendritic cells, T cells, Natural Killer cells ) to the site of infection – an activity that is also known as chemotaxis;
- lyse microorganisms by forming a terminal complement complex.
Both the protein crown and the immune system influence the biological fate of nanocarriers.
Nanocarriers for intravenous administration
Nanocarriers can be designed to stimulate or suppress an immune response. Stimulating the immune system is useful in vaccine development while suppressing it is crucial in the treatment of inflammatory and autoimmune diseases [1].
There is also a third situation: nanocarriers designed to “escape” the immune system and remain in circulation for an extended period. This long circulation, in general, favors the increase of the target’s exposure to the drug when compared to the free drug. However, it is not always linked to the superior effectiveness of nanomedicine. In other cases, rapid elimination is convenient, for example in the development of nanocarriers for detoxifying patients.
Regardless of the objective of the nanocarrier, knowing the immune response that it arouses can be a great ally in the development of new nanomedicines.
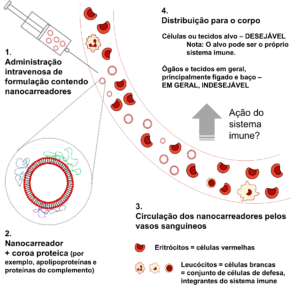
The destination of nanocarriers after entry into the bloodstream and interaction with the immune system. Upon reaching the bloodstream (1), the nanocarriers are covered by proteins (represented in blue, green, and purple) (2). The nanocarriers circulate through blood vessels (3), being distributed throughout the body (4) until their elimination. The circulation time and the distribution of the nanocarriers depend on several factors, one of which is the immune response.
Is the biological destination of the nanocarriers defined by the complement system?
Complement proteins are believed to be closely related to the circulation time of nanocarriers. Some authors also relate the complement to the hypersensitivity reactions observed in the clinic. The effect on pharmacokinetics and biodistribution, however, remains in debate.
- Polymeric nanoparticles
The work entitled “ Mechanistic understanding of in vivo protein corona formation on polymeric nanoparticles and impact on pharmacokinetics” [2], shows by studies in vivo that the complement cascade is not enough to explain the biological behavior of polymeric nanoparticles. Apolipoproteins J, on the other hand, have a protective effect, slowing the elimination of [2] nanoparticles. This work, published in 2017 by Prof. Dr. Nicolas Betrand (ULaval, Canada), by Profa. Dr. Eliana Martins Lima (UFG) and other collaborators raised new questions, among which I highlight: what is the effect of the complement cascade on liposomes?
- Liposomes
The liposomes are nanocarriers with wide use in the pharmaceutical area due to their biocompatible and biodegradable composition, among other advantages. They are formed by a bilayer of phospholipids, a structure similar to the animal cell. The surface of liposomes can be modified, for example, by the polyethylene glycol polymer (PEG). Liposomes covered by PEG are also known as PEGylated, while those without the coverage are called non-PEGylated.
There are several liposomes based nanomedicines approved by the FDA ( Food and Drug Administration ) and EMA ( European Medicine Agency ). Three major successes are:
- Doxil®/Caelyx®, a formulation containing liposomes PEGylated encapsulating the drug doxorubicin;
- Vyxeos®, a formulation containing non-PEGylated liposomes encapsulating the drugs daunorubicin and cytarabine;
- Myocet®, a formulation containing non-PEGylated liposomes encapsulating the drug doxorubicin.
Liposomes, both PEGylated and non-PEGylated,
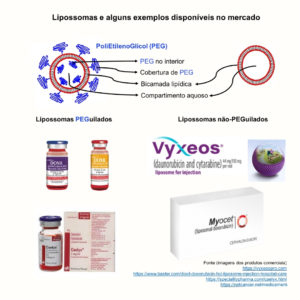
Some examples of commercial injectable liposome nanomedicines.
are weak activators of the complement cascade in vitro , as shown in “Role of the complement cascade in the biological fate of liposomes in rodents”[3]. This work informs us about the nano-bio interaction, without evaluating the application of the liposomes under study. Empty liposomes with different circulation profiles (fast and slow) were used. At first, these liposomes are applicable, by executing relevant modifications, for an immunostimulatory or immunosuppressive purpose.
In this work, it was shown that the complement cascade does not induce the release of liposome content when incubated in mouse, rat, or human [3] serum. Similar to what was observed with nanoparticles in the previous study [2], the impact of complement on the elimination of liposomes is subtle, being noticeable only in the late phase of liposome circulation when low doses were administered intravenously in mice and rats [3]. Under these conditions, the complement proteins guided the liposomes for distribution to the splenic B cells [3]. Another interesting result points out that the cellular composition of the spleen varies according to the immune condition of the mouse: with or without normal levels of complement proteins [3].
Perspectives
In conclusion, the effects of the immune system interaction with nanocarriers depend on several factors, such as the characteristics of the nanocarrier (type and composition), route of administration (including dose level and dosing system), and the immune condition of the animal (in our example, serum levels of complement proteins). We are on track to scale the effects of the crown protein and the immune system on the biological destination of nanocarriers. However, it still lacks a deep understanding of this dynamic process. Greater understanding will make us more assertive in the development of new nanomedicines.
Bibliographic references
1 Zolnik BS, Gonzalez-Fernandez A, Sadrieh N, Dobrovolskaia MA. Minireview: Nanoparticles and the immune system. Endocrinology 2010; 151: 458-65.
2 Bertrand N, Grenier P, Mahmoudi M, Lima EM, Appel EA, Dormont F, et al. Mechanistic understanding of in vivo protein corona formation on polymeric nanoparticles and impact on pharmacokinetics. Nature Communications 2017; 8: 1-8.
3 Viana IMO, Grenier P, Lima EM, Bertrand N. Role of the complement cascade on the biological fate of liposomes in rodents. Nanoscale 2020; 12: 18875-84.
Escrito por Iara Maíra de Oliveira Viana, PhD in pharmaceutical nanotechnology from UFG.